Albert Group Research Projects
Polymer Crystallization | Polyorganosiloxanes | Block Copolymer Blend Morphology | The Effect of Polymer Architecture on Self-Assembly of Block Copolymer
Polymer Crystallization
Crystalline polymers are those that, because of their simple backbone structure as well as favorable interchain or intrachain interactions, are able to form locally ordered regions both in the bulk and in thin films. This gives crystalline polymers certain favorable characteristics over amorphous materials, such as material toughness and varying levels of opacity that are useful for optical applications. Crystalline polymers include: poly(ethylene glycol) (PEG), poly(caprolactone) (PCL), polyethylene (PE), polypropylene (PP), and isotactic polystyrene (PS).
Of specific interest are crystalline polymers that also exhibit biocompatibility and biodegradation, such as polycaprolactone. The crystallinity of this material extends its biodegradation time and makes it an excellent choice for drug delivery and tissue engineering applications. Beyond that, PCL is a model polymer for the study of not only bulk crystallization characteristics, but also the still poorly understood thin film crystallization seen in the single to tens of nanometers range, and crystallization in polymer blends.
Polyorganosiloxanes
Polysiloxanes are a class of organosilicon molecules containing, at the very base, the silicon-oxygen-silicon linkage. Different from the typical organic polymers with a carbon-carbon or carbon-oxygen backbone, the polysiloxane backbone affords these materials with low thermal conductivity, high thermal and chemical stability, gas and solvent selectivity, and molecular flexibility.
Polydimethylsiloxane (PDMS) has been the most widely used polysiloxane because of its relative ease of synthesis, chemical inertness and biocompatibility, and its ability to form mechanically tunable crosslinked gels and elastomers. It is used in the fields of biomimetics, microfluidics, bioengineering, membranes, and alternative energy. That being said, the world of polysiloxanes is a large one, and various useful materials can be created based off this simple backbone architecture. Polyvinylmethylsiloxane (PVMS) is a similar siloxane analogue to PDMS, but with an easily modifiable pendant vinyl group. The ease with which researchers can, via thiol-ene chemistry, link a wide variety of chemical handles to this backbone and by association, the material surface, has provided a material useful for cell studies, membrane fabrication, and the creation of robust microfluidic devices.
Block Copolymer Blend Morphology
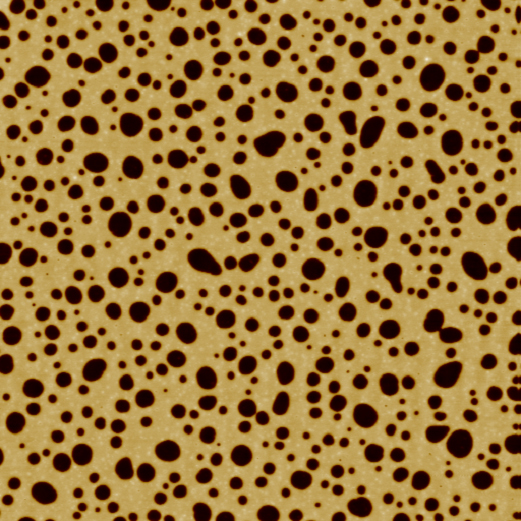
Nano- and micro-structured materials are important in templating and membrane applications, which require thin films with well-defined morphologies on application-specific length scales (e.g., peptide arrays ~nm, cell arrays ~μm). In order to understand how to take advantage of self-assembly processes to access length scales spanning multiple orders of magnitude, this project will systematically investigate the fundamentals of block copolymer- homopolymer blend phase separation in composition regimes that lie between the nanostructured assemblies of homopolymer-swollen block copolymer domains (~10-100 nm) and the mesoscale morphologies of block copolymer compatibilized blends (~1-100 μm). In these blends, introducing curvature constraints through asymmetry in the block copolymer composition will open up a rich phase space for self-assembled, periodic, nano- and micro-structured materials.
The first part of the project will focus on bulk mixtures and assessing the extent to which the preferred block copolymer curvature is capable of maintaining structural periodicity, which is absent in homopolymer blends. The second part of the project will examine how thin film confinement changes the blend morphology as compared to the bulk, since many applications of these materials will require their use in thin film geometries. The objectives for both aspects of the project are designed to seek fundamental scientific understanding of polymer self-assembly processes while simultaneously identifying related research areas.
The Effect of Polymer Architecture on Self-Assembly of Block Copolymer
The goal of our research is to systematically investigate the effects of different polymer architectures on the self-assembly of block copolymers in thin films (~10-100 nm). Numerous research efforts have been conducted on individual characteristics such as block copolymer architecture, polymer blends, and interfacial tension. Few publications have explored the relationship between these three effects on block copolymer self-assembly. Instead of focusing on diblock structures, in which a plethora of research has been conducted, our aim is to contribute to the field of multi-block and non-linear polymer architectures.
Cyclic and star block copolymers will be synthesized for use in our studies of the effect of polymer architecture on self-assembly. Cyclic block copolymers exhibit unique physical properties compared to their linear counterparts. Such properties include a smaller hydrodynamic volume and domain spacing, due to conformational restrictions. The decrease in domain spacing can allow for easier control of lithographic techniques, especially in the sub-20 nm size regime, a regime in which lithography faces difficulties and high costs. Additionally, star block copolymers exhibit unique topological structures and attractive physical/chemical properties. Star block copolymers can be used for stimulus-responsive materials because of their high density functionalities, drug delivery vessels in the form of stable micelles, and cost-efficient dispersants in oil spill recovery.